03/10/2015
Last month's column (Pumps & Systems, February 2014) reviewed the pump selection process and described how many users oversize pumps by adding design margins to account for unknown conditions present during the pump specification process. Design margins are similar to insurance, providing protection for unknown conditions expected in the life of the plant. This column will explore how oversizing a pump affects the motor driving the pump. It will also examine the adverse effects that occur when a pump is no longer operating at its best efficiency point (BEP) for extended periods of time and situations in which a design margin increases cost of ownership.
Drive Operations
Induction motors used to drive pumps are efficient in converting electrical energy into mechanical energy. A motor is 92 to 96 percent efficient depending on its classification and frame size. The efficiency is relatively constant from 50 to 100 percent of full load, with only a slight drop off when operating down to 25 percent of full load. Figure 1 shows a typical efficiency graph for a totally enclosed fan-cooled (TEFC) motor at a range of sizes operating at varying loads.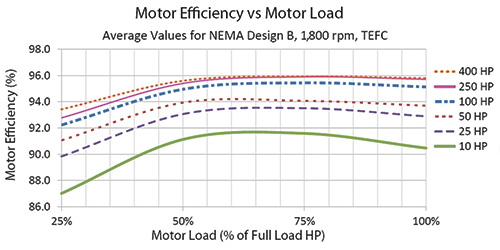
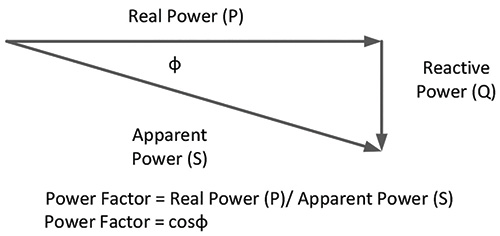
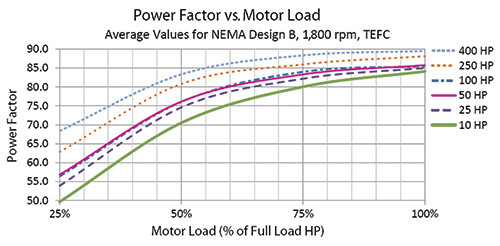
Pump Operations
A pump is designed for a specific range of flow rates and head values to meet the needs of the market. The pump's peak performance and maximum service life for flow rates will occur around the BEP. A pump operating at maximum efficiency results in a uniform flow of fluid through the pump. Figure 4 shows the pump curve used in last month's column. The pump's BEP of 79 percent occurs at 1,070 gallons per minute (gpm). In addition, the efficiency value quickly drops off from the BEP to the minimum and maximum recommended flow values. Unlike a motor, the pump operates away from its BEP. The flow through the pump is no longer uniform, resulting in recirculation within the impeller. This non-uniform flow creates uneven pressure distribution in the pump, causing an increase in vibration and hydraulic load as well as a reduction in efficiency. The price here results from a combination of energy costs, mechanical wear and operational inefficiencies. In Figure 4, the process flow rate through this pump for the first year is only 200 gpm, with the flow rate increasing to 400 gpm in the second year and 800 gpm by the fifth year. The design flow rate of 800 gpm is only 75 percent of the pump's BEP flow.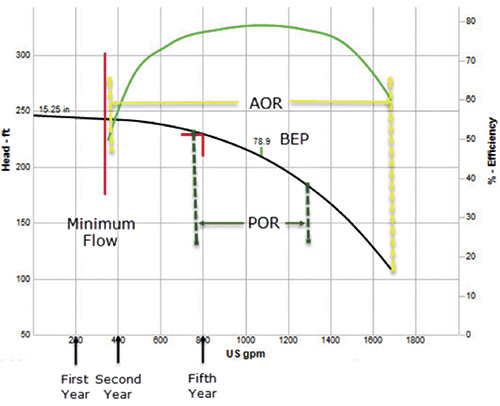
Evaluating the Pump
In Figure 4, the 200 gpm of flow rate for the first year of operation is well below the minimum AOR flow rate of 350 gpm recommended by the manufacturer. If the pump were to operate at this flow rate for an extended period, extensive mechanical degradation would occur. To achieve the manufacturer's minimum flow of 350 gpm, a recirculation line will need to be installed to recirculate 150 gpm back to the supply tank. When the preliminary sizing was performed, design margins for pump head were added. Unknown conditions were factored into the preliminary design including the elevation of the tanks, tank levels and pressures, pipe length, and number of valves and fittings. Now that the system is built, the conditions are known. In Figure 4, the pump head at the design flow rate of 800 gpm was calculated at 211 feet, but the system as built only requires 190 feet of head. The additional 21 feet of head required by the design margin is excess head. Because the full value of design margin is not needed and the pumps are already in place, the additional head (the 21 feet) is absorbed across the control valves to eliminate excess energy.Assessing the Cost of the Piping System
As demonstrated, assigning design margins of head and flow are an integral part of every pump selection. They provide designers and engineers with the required flexibility to select long lead items early in the design process. Just like any expense, the amount of insurance and resulting costs should be evaluated and re-evaluated. Oversizing pumps increases the system's operational, maintenance and capital cost. Without knowing the true cost of owning and operating a pump, the process cannot be improved. The purpose of the pump system assessment is to review how the installed and operating system is performing. The system is reviewed, and margins are adjusted for optimization. The initial design phase can only supply a best-case scenario. Determining costs associated with each component in a piping system helps calculate actual operating costs, and performing a post assessment allows engineers and operators to evaluate what can be done to improve system performance further in full operation.
References
Welcome to the Hydraulic Institute. (2014, January 1). Retrieved January 7, 2015, from http://www.pumps.org/content_detail.aspx?id=4330