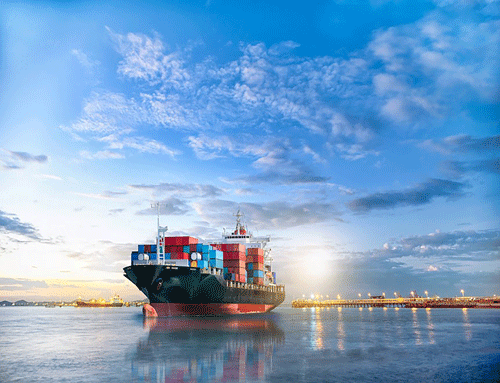
Methanol (CH3OH) is a colorless liquid that boils at 64.96 C (148.93 F) and solidifies at −93.9 C (−137 F). For many decades, methanol has been widely used in chemical processing as a building block for thousands of products such as resins, glues, fabrics and pharmaceuticals.
Upon its discovery, methanol was initially produced by distilling wood. Over the years, steam methane reforming (from coal or natural gas) has been used to create a synthesis gas that is fed into a reactor with catalysts to produce methanol. Modern methods continue to evolve to produce methanol via greener processes—namely the combination of biogenic carbon dioxide (CO2) and hydrogen (H2) in the presence of a catalyst.
Methanol has high-octane properties. It works with many existing engine types as a drop-in or dual fuel. Back in the 1990s, methanol was marketed as an alternative fuel for compatible vehicles. At its peak, nearly 6 million gallons of blended methanol and gasoline were used annually in the United States.
In 2015, methanol witnessed a resurgence in the maritime industry where it is used to power vessels such as tugboats, ferries and industrial lift/multipurpose ships. Over the next few years, maritime OEMs began making methanol-compatible engines. In 2022, large industrial shipbuilders began taking orders for new dual-fueled cargo ships. There are almost 100 different harbors around the globe with the infrastructure needed to supply methanol for maritime bunkering. The maritime industry sees methanol as one of the leading solutions for decarbonization. Carbon-neutral e-methanol can be produced from green hydrogen (made via electrolysis powered by wind or solar) and CO2 from industrial flue gas, or from biogenic feedstocks like agricultural waste, landfills or dairy farms.
In 2022, a logistics and supply chain services company announced a program to use green e-methanol for one of its large container vessels. They are working with an energy company to build an e-methanol facility that will eventually produce
10,000 tons of carbon neutral e-methanol—all of which will power a single cargo ship. The methanol facility will use green hydrogen from a 50-megawatt (MW) electrolyzer (powered by solar) and biogenic CO2 to produce the fuel. This program could become a blueprint for how to scale green fuel production by collaborating with several partners across the ecosystem.
One of the partners in this project is an equipment manufacturer that makes centrifugal gas compressors used to blend H2, CO2 and catalysts together. This process involves a carefully orchestrated series of gas feeding loops at particular flows and pressures that are needed to make the e-methanol fuel.
Criteria for Selecting Compressors Used in E-Methanol Production
Here are 10 requirements that were evaluated in selecting the compressors used to handle H2 and CO2 to make e-methanol.
Safety: Based on decades of experience in traditional oil and gas processing industries, today’s compressors are specifically designed to handle flammable gases. Sealing systems minimize leakage, and monitoring capabilities continuously analyze the behavior of the dry gas seals.
Speed and performance: Integrally geared centrifugal compressors use a gearbox to increase the speed from the driver to the impeller(s). The pinion shaft in the gearbox can be sped up to 42,000 rotations per minute (rpm) to match the optimal impeller geometry. A variable speed drive system facilitates a wider range of operating conditions to deliver the necessary flow and pressure.
Flow and pressure capacities: Like many other applications, low-flow and high-head capabilities are important when it comes to making e-methanol. The compressor casings used in this application have a max capacity of 18,000 cubic meters per hour (m3/hr) and a design pressure up to 350 bar.
Efficiency: When it comes to operational expense (opex), efficiency is paramount. The compressors used for this application feature open-type impellers and aero-end static and rotating parts that were designed using computational fluid dynamics (CFD) tuned to the specific conditions at the site.
Hydrogen purity: These compressors use dry gas seals that provide contamination-free compression, meaning the gas exits the compressor as pure as it enters. The oil lubricating the gearbox internals is kept away from the process gas by an inert gas purged separation seal. All the sealing elements prevent ingress of external gas or liquid into the compression cavity.
Small footprint: Compressor skids can be large for multistage compressors. The unit selected for this project was a single stage, integrally geared compressor that could deliver the required processing power in a smaller footprint, which saved space in the processing area and reduced weight and vibration (compared to a multi-stage or reciprocating alternative).
Simple maintenance: The architecture of integrally geared compressors allows access to impellers and dry gas seals without removing the gearbox components. The bearings can be accessed without disturbing the process piping or the aero-end components. The high-speed shaft components can be replaced/reassembled without lifting devices and without the need for heating, shimming or rebalancing, which simplifies maintenance.
Reliability/uptime: This application requires multiple catalyst reaction modes (activation, flushing, etc.) which require different gas composition within a range of megawatts (MW) from 2 to 29 mass in grams of one mole (g/mol), mixing H2, nitrogen, O2 and CO2. The compressors used in this project can provide an uninterrupted service range between three and seven years, delivering the uptime needed to produce e-methanol without requiring a spare/standby compressor.
OEM experience: There are thousands of centrifugal compressors deployed in refineries, petrochemical plants and power generation facilities. These machines have been safely handling H2 and CO2 for decades. This experience has been codified in recognized norms, including some of today’s American Petroleum Institute (API) standards. It also helps to guide aftermarket services when it comes to spare parts, rerates and predictive maintenance.
Total life cycle costs: The predictable profit margins associated with traditional fuels have yet to be established for today’s new energy solutions. Because of this, everyone in the new energy space is mindful of capital expenditure (capex). Lower installation costs, high efficiency, three to five years of uninterrupted service and streamlined maintenance are all requirements for equipment used in delivering competitive greener products.
Today, everyone in every industry seeks ways to reduce their carbon footprint. There are more than 60,000 commercial vessels roaming the oceans, accounting for almost 5% of global greenhouse emissions annually. One of the primary goals of the International Maritime Organization is to cut the shipping industry’s greenhouse gas emissions in half by 2050 (compared to 2008 levels). Replacing traditional bunker fuel with greener alternatives, such as e-methanol, is a key step for this energy transformation.
All of the players involved in this ecosystem—shippers, ship builders, energy producers and the equipment manufacturers that support these players—are all collaborating in new and exciting ways to create a value chain that will scale green fuel production exponentially in the years ahead.