I have been writing “Common Pumping Mistakes” for Pumps & Systems for more than three years. Typically the hardest part of the job is topic selection so it will be fresh, educational and interesting. This month, I am writing on a collection of shorter subjects and baking them up into one article. Instead of a meal, we will have hors d’oeuvres. Hopefully it will satisfy your appetite. If you have been reading my column, many of these tidbits will be a review. These comments are based on single-stage overhung centrifugal pumps moving ambient temperature clear water, except when otherwise noted. Pumps are really designed to operate at only one point. That hydraulic condition of one point of head and flow is the best efficiency point (BEP), also known as the best operating point. Anywhere else on the published set of curves is simply a commercial compromise. It would be too expensive for most end users to have a pump designed and built for their unique set of hydraulic conditions.
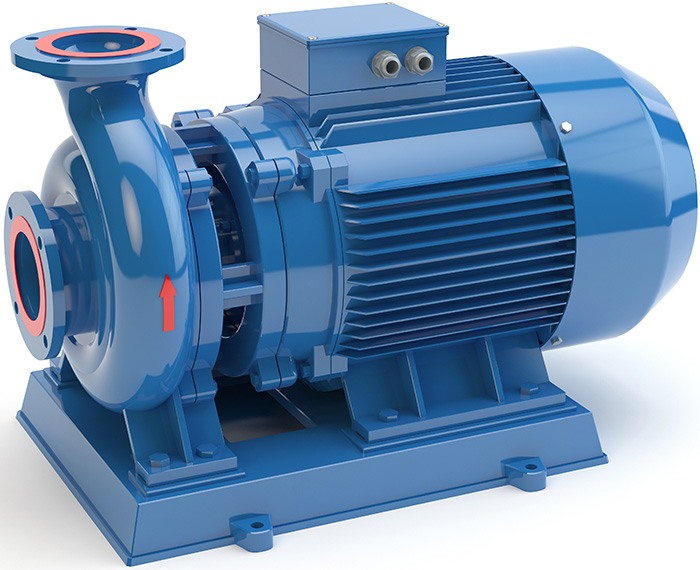