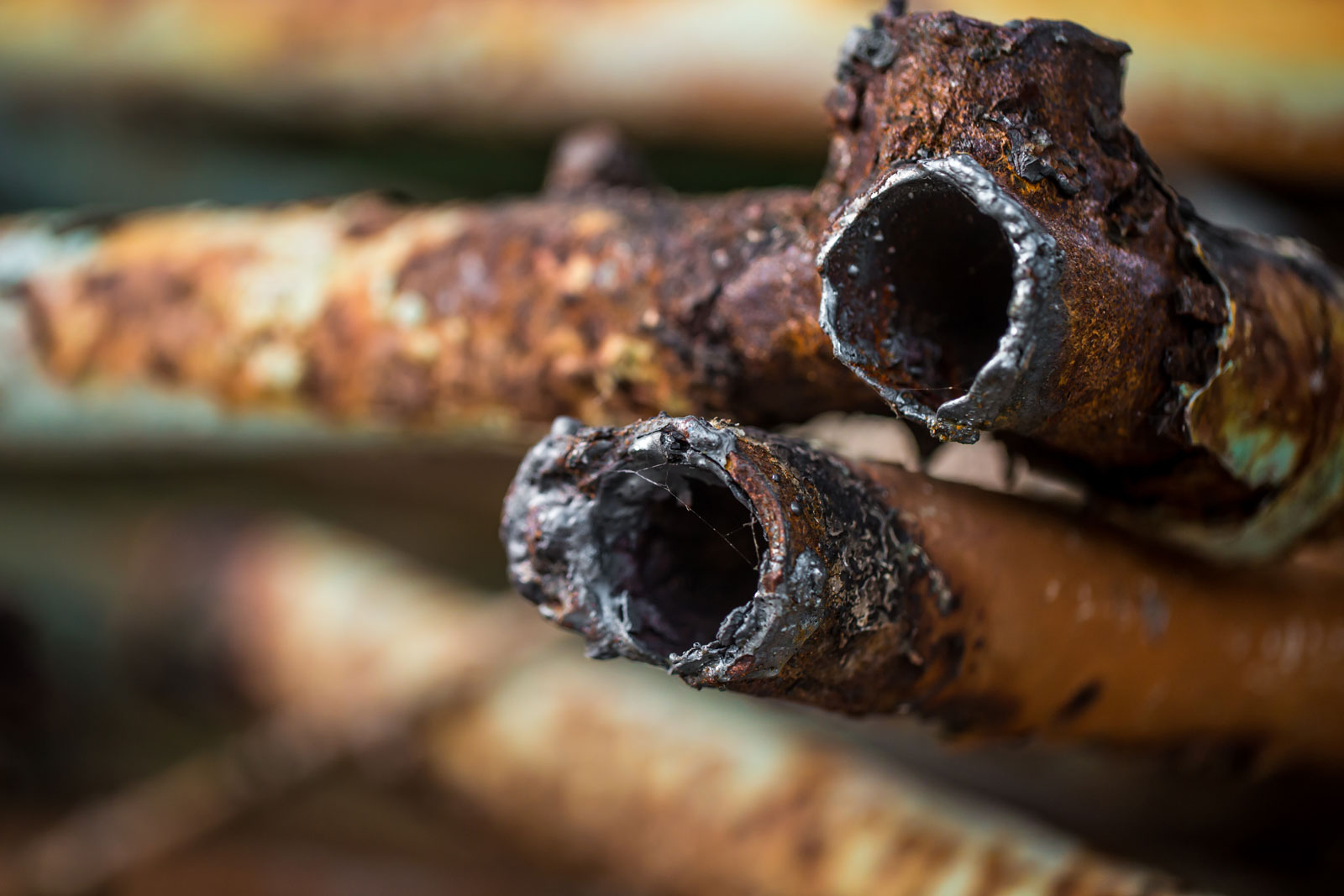
The benefits of insulating tanks, process equipment and pipework to reduce energy usage and the risk of personal injury by burns cannot be overstated. An unfortunate downside, however, is that if corrosion of the underlying steelwork is initiated, it often proceeds undetected until a dangerous loss of wall thickness results or, even worse, catastrophic failure occurs, leading to loss of containment. Corrosion under insulation (CUI) is, therefore, a major concern to facility maintenance engineers.
Steel will corrode when it is in contact with water and oxygen, so if water can penetrate the insulation, corrosion will likely result. Examples of possible causes include:
- Poor choice of insulation—material such as mineral wool, fiberglass and calcium silicate can absorb water from rain, deluge systems or leaks in the system. In addition, certain insulation materials contain chlorides, which will accentuate galvanic corrosion and induce stress corrosion cracking of austenitic stainless steel.
- Poor design or installation of the insulation, especially in problem areas such as cut-outs for nozzles, valves and flanges, etc.
- Mechanical damage to insulation as part of plant operation or maintenance activities
- Insulation not being correctly replaced after inspection or maintenance activity
- Degradation of sealants by natural weathering, thermal oxidation or ultraviolet (UV) degradation
- Water condensation, particularly on refrigerated or chilled water systems and hot surfaces when there is temperature cycling
- Absorption of airborne moisture in factories where steam is predominantly the heating medium
In addition, other factors might influence the likelihood of corrosion, such as contamination from salts in the atmosphere, particularly in marine environments, or leakage of process fluids and acids.
Fortunately, many coating systems on the market can help to prevent CUI by providing an effective barrier against corrosion or chemical attack. Thermally sprayed aluminum (TSA) or polymeric coatings are commonly used and can be effective, but product selection to meet specific process conditions is important.
In the case of polymeric coatings, epoxies and epoxy novolacs are the most commonly used as they offer the greatest formulation flexibility. By the appropriate choice of resins, hardeners and fillers, coating materials can be created to cater to a wide range of design criteria, such as the ability to be applied onto poorly prepared or damp surfaces, high temperature resistance, low temperature flexibility, chemical resistance, etc., while at the same time protecting the steel substrate against corrosion. Epoxies and epoxy novolacs are widely recognized as providing excellent adhesion and can typically be used at temperatures down to -4 F/-20 C and, in the case of novolacs, are thermally stable and, when properly formulated, can retain a high percentage of their performance properties up to around 446 F/ 230 C. Most commonly, they are formulated as two-component systems but single-pack, heat-activated latent hardener systems are also available. More recently, there has been increasing use of silicone systems for both low temperature (-76 to -112 F/-60 to -80 C ) and high temperature (932 to 1,112 F/500 to 600 C) situations. In addition, recent developments have seen the introduction of novel low thermal conductivity coatings, which not only reduce surface temperature and heat loss but also provide protection against CUI. Depending on performance and design requirements, these materials can be used at thinner coating thicknesses as a CUI coating, which will enhance the performance of conventional insulating systems, or can be used at higher thicknesses as a stand-alone system to reduce heat loss and surface temperature.
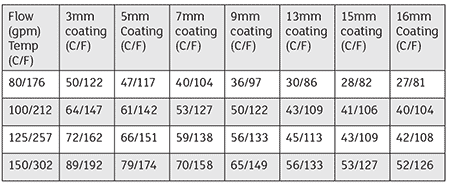
The effect of coating thickness on temperature reduction is illustrated in Image 2, which compares the surface temperature of a steel panel coated in various thicknesses of a thermally insulating barrier coating when the underlying steel is heated on a hot plate.
The results demonstrate the ability of the material to effectively reduce the surface temperature of the coated panel. First-degree (minor) burns are generally regarded as occurring at 140 F/60 C when contact is short and 122 F/50 C when contact is held. Therefore, even at hot plate temperatures as high as 302 F/150 C, the surface temperature of the coated panel can be reduced to less than 140 F/60 C using an appropriate thickness of coating. However, the low heat capacity of the experimental material means that even at lower coating thickness and higher surface temperatures, it is still safe to touch the surface without burning.
This is because the amount of heat contained in the coating is too low to cause burning and the low thermal conductivity of the coating ensures that it cannot be transferred quickly enough to fully replenish any heat transferred to the skin. For example, in this testing it was found that even when the 3-millimeter (mm) section of the coating was touched when the sample was on the hot plate at 302 F/150 C, no discomfort to the skin was detected even after several seconds of contact. This difference between measured temperature and touch temperature is the subject of independent studies and, because of this ability, in many cases the use of such coatings can eliminate the need for insulation or safety caging if safety is the only consideration.
With the ever-increasing cost of energy and associated environmental considerations, however, reducing heat loss is another important consideration to industry and is a key driver for equipment to be insulated in such a way without the risk of CUI. By using such materials, the energy savings can generally pay for the cost of installation of the system many times over the lifetime of the equipment.
Using finite element analysis (FEA), the potential energy savings as a result of using a thermally insulating barrier coating system have been quantified. This study also compared temperature and heat losses with those of a more conventional insulating material: rockwool.
An 8-inch shed 40 stainless steel (304) pipe was used in this example. To compare the insulated and noninsulated pipe, three individual pipe models were designed and submitted to the same thermal constraints as follows:
- Heat load: 356 F/180 C was applied to the inner surface of each three pipes
- Convective losses: 7 watts per square meter kelvin [W/(m2K)] at an ambient temperature of 68 F/20 C was applied to the external surfaces of the three pipes
- Radiative losses: it was assumed that all three pipes were of the same external surface emissivity (0.95) with a surrounding temperature of 68 F/20 C
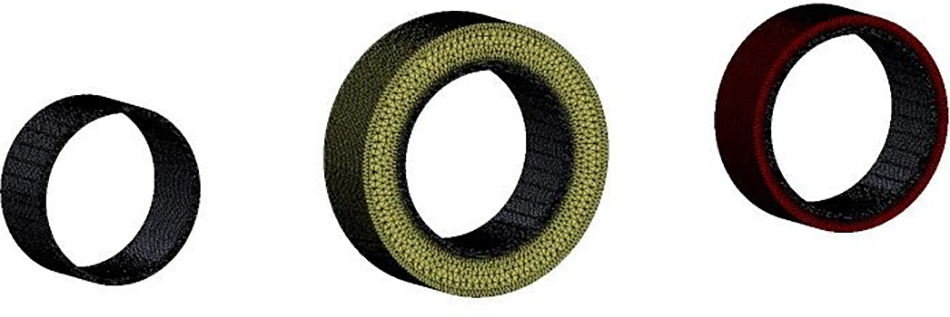
Image 3 shows the three-pipe system and the FEA mesh representation. Each pipe is discretized into subelements that are used for each calculation point.
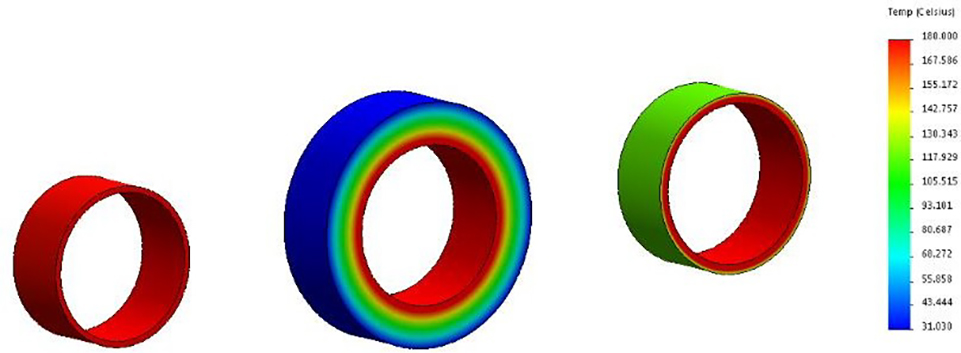
Image 4 illustrates the results of this analysis. With 5 mm of the thermally insulating barrier coating, the surface temperature is reduced by 105.1 F/58.4 C and the total heat loss is almost halved. As expected, the surface temperature and heat losses are lower when using 10 times the thickness of rockwool, but the thin layer of the thermal barrier coating still almost halves the heat loss from the pipe while providing corrosion protection and eliminating the risk of CUI.
Furthermore, by increasing the thickness of the thermally insulating barrier coating from 5 mm to 15 mm, both the surface temperature and heat flux can be further reduced by 34.3% and 45.3% respectively.
Furthermore, rockwool can soak up moisture from the environment leading to it becoming more thermally conductive and more likely to promote CUI problems. Several studies show that the thermal conductivity of “wet” rockwool can increase up to 0.125 W/(m2K).
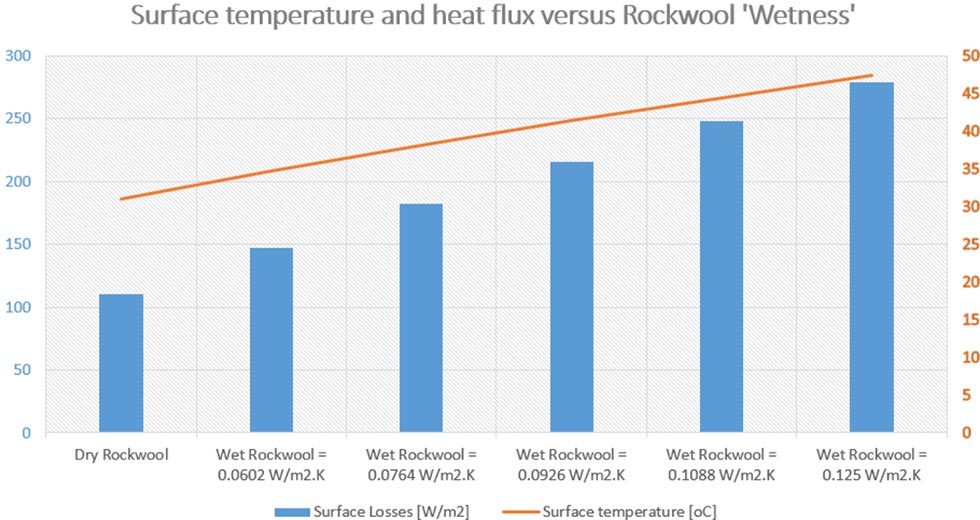
The graph in Image 5 shows the result of a study where the thermal conductivity of rockwool was increased from dry (0.044 W/(m2K)) to wet (0.125 W/(m2K)).
Here, there is an increase in heat loss and surface temperature as the degree of moisture in the rockwool increases while the nonporous epoxy-based thermal barrier coating does not suffer this disadvantage.
In conclusion, epoxy-based coatings provide an effective way of protecting steel structures against corrosion under insulation. Additionally, the advent of low thermal conductivity barrier coatings means the need for additional insulation can, in some cases, be eliminated. If it is required, its performance is enhanced and the substrate is protected against CUI problems. The formulation flexibility of epoxy-based coatings means that such materials can be tailored to meet a wide variety of application and service conditions and ongoing developments are targeted at providing further enhancements to thermal barrier protection.
Acknowledgment
The authors would like to thank Dr. Francois Pierrel of EnerTherm Engineering for the finite element analysis.