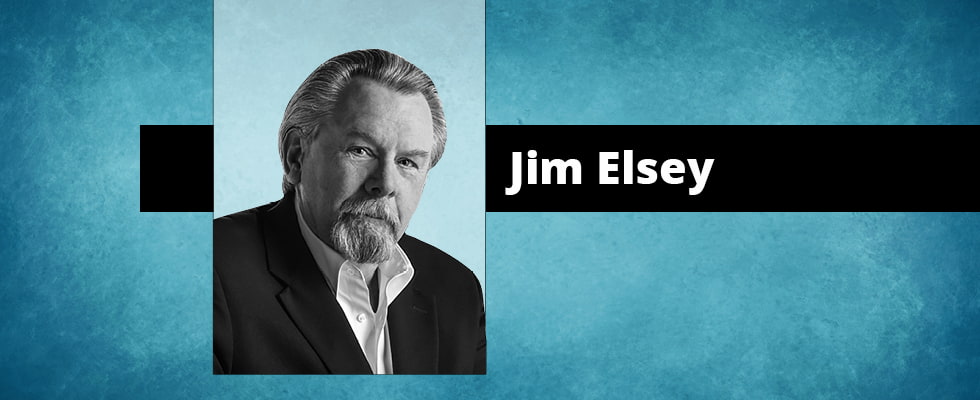
With a whimsical nod to the “Game of Thrones” fans out there, winter is coming soon, and this October title was chosen for two reasons. One is a simple reminder to those of us that have yet forsaken proper freeze protection for pumps, pipes and other components in our systems. Second reason: Why did I really invoke the title, “Winter is Coming?” During the course of my work and due to my association with the ASM International (formerly known as the American Society of Metals) and American Society of Mechanical Engineers (ASME), I have been involved with the cryogenic treatment of steel components as it relates to the life extension and reliability of pump parts. The extraordinary low temperatures required for these processes (negative 310 F) always reminds me of winter.
Cryogenics
Cryogenics relates to the production and behavior of materials at very low temperatures. What do golf clubs, gun barrels, guitar strings, saxophones, helicopter gears, racing engines, pump parts and NASA spaceships all have in common? They can all be distinctly better when cryogenically treated. Why has “Swiss steel” been so famous for quality for the last 100 years? Is it because they post-treat the metal in a cold environment? I am not 100 percent sure, but the default (post-production) cold treatment provided by the geographical position in the cold Swiss Alps is one likely reason. Cryogenics is not to be confused with cryonics, which is the pseudoscience of freezing human bodies, most notably the famous baseball player Ted Williams. Cryogenic metal treatment has been around for approximately 100 years, but it was not until the mid-to-late 1960s that significant progress was made in the commercial processes to make it economically viable. In the science world of cryogenic treatments, it is common to use the Kelvin scale (K) for temperatures or sometimes Celsius (C), but for this article and reader familiarity we will use Fahrenheit (F). We will not use the Rankine scale even though it relates well to the Fahrenheit scale. The cryogenic temperature range is normally defined by the National Institute of Standards and Technology (NIST) as ranging from minus 238 F down to minus 460 F. Note that it is not possible to be colder than 0.0 degrees Kelvin, which is absolute zero.Why Use Cryogenic Treatment
Not all types of metal can be treated successfully, but for the ones that can, the three main reasons are:- Measurable improvement in wear resistance. It is not uncommon to improve wear resistance of parts by 30 percent and in some cases much more. Many steel parts (especially tool steels) experience an increase of more than 200 percent.
- Improved durability because of fewer imperfections in the steel structure. This is due to an improved and consistent grain structure in the metal.
- Stress relief. When metal cools down from the liquid (molten) state to a solid, there is always some amount of residual stress. Eliminating those stresses makes the part more reliable because mitigation of the residual stress will result in a direct reduction of fatigue failures.
For the Metals & Technical Folks
Steels can appear in many microscopic structures and forms that include:- ferrite (also known as alpha iron)—a body-centered cubic structure
- austenite (also known as gamma iron)—a face-centered cubic structure
- martensite—a steel structure obtained only when austenite is suppressed down to a temperature where it is forced to transform to a body-centered tetragonal