HI Pump FAQs is produced by the Hydraulic Institute as a service to pump users, contractors, distributors, reps and OEMs.
05/20/2015
Q. What principles drive the pumping action in a controlled-volume metering pump? A. The pumping action is developed by a reciprocating piston. This reciprocating motion develops a flow profile represented by a sine wave. Actual rate of flow is determined by the following formula: Rate of flow = Displacement x Cycles per unit of time x Volumetric efficiency Figure 7.3.1a illustrates how changes in discharge pressure minimally affect the rate of flow from a reciprocating, controlled-volume metering pump.
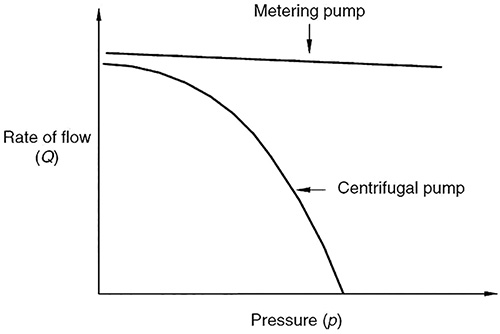
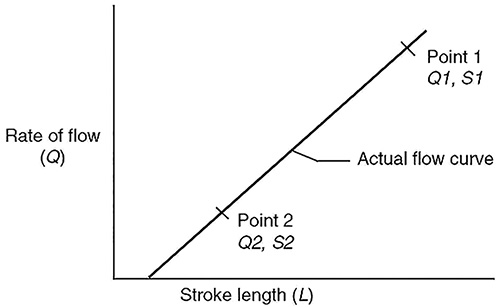
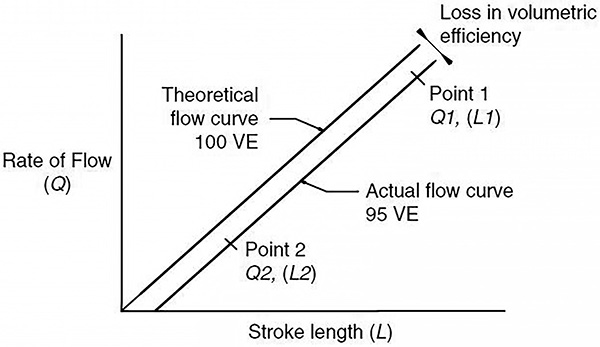
- Emission control of process fluid to the environment under normal operating conditions
- Emission of process fluid under upset conditions or failure of the primary seal
- Acceptable life (time between repairs) as a result of inadequate lubricating properties of the pumped medium, which may be a result of too many solids too close to vapor pressure, too low viscosity in combination with pressure and speed, or too high pressure differential for one set of faces
- Dual pressurized seals can seal products that crystallize, burn or are otherwise unsuitable for single seals.
- Dual seals can limit loss to the atmosphere. This feature may be a factor in seal selection for equipment handling hazardous materials.
- Seal life may be improved as the seal faces operate in a clean, cool environment.
- Seal performance can be confirmed by monitoring the barrier fluid.
- The faces are lubricated using a clean, cool liquid—usually oil, water or glycol—which provides low wear rates.
- Dual gas seal faces are separated with a clear inert gas such as nitrogen.
- The seal operates independently from the pump. Consequently, loss of product, cavitations or other disturbances can be better tolerated.
- Zero emissions or product leakages are released into the atmosphere.