In my June column on pump and motor testing, I said that three-phase voltage variation and unbalance can have a significant effect on motor insulation life. Voltage variation is defined as the difference between the motor nameplate voltage and the incoming source voltage. This assumes that all three phases are the same voltage.
Variation can be either negative or positive. Unlike variation, voltage unbalance refers to the differing voltages that can occur in each of the three phases. This month’s column reviews voltage variation. Next month’s column will cover voltage unbalance.
Low-Voltage Motor Requirements
Prior to the introduction of the T-frame motor in 1964, low-voltage motors were designed to operate at 220 and 440 volts. Today, that standard is 230 and 460 volts and is referred to as the utilization voltage (American National Standards Institute C84.1). These voltages were selected because they reside in the middle of the service range that a utility is required to supply. They also allow for the voltage drop that can occur because of the distance between the motor and the service entrance.
The National Electrical Manufacturers Association (NEMA) standards recommend that motors should be designed to operate satisfactorily at a voltage variation of plus or minus 10 percent. For 230 volts, that range is 207 to 253 volts; for 460 volts, it is 414 to 506 volts. The word “satisfactorily” is important because parts of this range can be less than optimal.
Figure 1 shows the effect of voltage variation on several motor performance characteristics. It can be used to show the general effects, but variations will occur based on a particular motor design. The intersection of the zero points on the x- and y-axes represents the motor nameplate voltage.
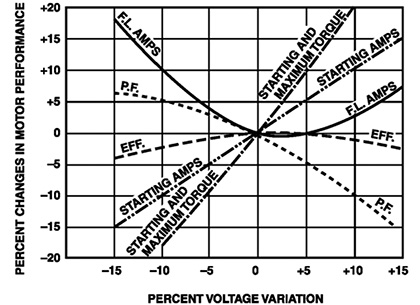
Low-Voltage Effects
The left side of Figure 1 shows the low-voltage effects. A 10 percent reduction in nameplate voltage reduces the motor efficiency by about 2 percent. In most cases, this will convert a more costly, premium efficiency motor to the older, 1995 EPACT design. That same voltage reduction will increase power factor by 5 percent. This is a good thing, but there is a more efficient way to achieve improved power factor. Reduced voltage has its largest effect on motor torque. As shown, a voltage reduction of 10 percent will result in a torque reduction of about 19 percent. This is not usually a concern with variable torque machines, such as centrifugal pumps and fans. It could, however, be a problem with constant torque loads.
The most important characteristic is full load current at lower voltages. As shown in Figure 1, its increase is almost directly proportional to the reduction in voltage. A voltage reduction of 10 percent will increase the full load current by 10 percent. This is not unexpected because the power in watts is proportional to the product of the voltage and amps. This is not a problem if the measured amperage remains below the nameplate, full-load amperage. If, however, the motor is loaded close to the nameplate horsepower, the amperage will exceed the nameplate maximum. This is not necessarily a problem if the 10 percent reduction is brief because the motor’s service factor is designed to handle intermittent voltage drops. If it is constant, the motor service factor is eliminated, and any additional voltage drop will increase the operating temperature. The result will be a reduction in insulation life.
When 230-volt motors are operated on 208-volt power systems, the normal 15 percent service factor disappears, and additional voltage drops usually cannot be tolerated. Options include derating the motor horsepower, upgrading to the next higher horsepower or using a motor wound for 200 volts.
The final characteristic is starting amps. The opposite occurs when the motor starts, and starting amps are reduced with a reduction in voltage. This is the basis for wye/delta and solid-state, reduced-voltage starters.
High-Voltage Effects
An increase in supply voltage above the motor nameplate rating has the opposite effect of low voltage for most motor characteristics. However, two exceptions exist. The first is efficiency. It is also reduced at higher voltages, but not as much as it is at lower voltages.
The second is full-load amps. Full-load amperage remains steady with small voltage increases but can increase rapidly above 5 percent. For many motors, the full-load amp curve is much steeper than the one in Figure 1.
Why do amps increase with a rise in voltage? Unfortunately, this is not a simple power issue: It is related to the magnetic field produced in the stator. At normal voltages, the motor windings create magnetic fields in the stator laminations. As voltage increases, these fields can become saturated. Once saturated, the field cannot increase in intensity, so the motor draws more current in hopes of overcoming the saturation. The onset of saturation does not depend on motor loading. It will also occur in lightly loaded motors. The higher current caused by saturation increases the operating temperature and reduces insulation life. Fortunately the newer, premium-efficiency designs are somewhat more tolerant of high voltage than older designs.
Torque undergoes a major increase with a rise in voltage, and so do the starting amps (inrush current). Therefore, close attention must be paid to the number of starts per hour when operating at higher voltages. Remember, inrush current can be five to seven times the full load current. High voltage also causes a major reduction in power factor, which will increase circuit loading and possibly anger the utility.
The plus or minus 10 percent voltage tolerance recommended by NEMA should not be used continuously. It is included to accommodate the normal ups and downs of plant and utility supply voltage. If high-voltage variation (plus or minus) is continuous, correcting the variation is always best. Operating as close to the nameplate voltage as possible will maximize insulation life.