The old joke about falling from a tall building is that “it isn’t the fall that kills you, it is the sudden stop at the bottom.” When it comes to cavitation, it is not the formation of the vapor bubbles that kills the pump, it is the subsequent collapse. There are plenty of articles on net positive suction head (NPSH) and cavitation that talk about the bubble formation and the consequential pump damage in a broad sense, but the details of the damage mechanism are rarely discussed. The focus of this article is looking into why are we so worried about a few bubbles. Cavitation causes an increase in pump noise and vibration, but more importantly, a drop in performance, efficiency and impeller erosion. Not all of the damage from cavitation is metal loss or metal damage. Sometimes the issue is shortened bearing and mechanical seal life due to the unsteady flows (surging). Simply defined, “classic cavitation” from the perspective of centrifugal pumps is the formation of bubbles in the pump inlet near the eye of the impeller. The bubbles form because local pressure has dropped below the vapor pressure of the fluid (another way to view this is that the NPSH margin is not sufficient). Less than a fractional second later as the bubbles transit along the low pressure side of the impeller vanes, they enter a region of higher pressure and collapse. I refer to this as classic cavitation to differentiate it from other causes of cavitation, such as suction or discharge recirculation that manifests on the other side of the impeller vane. Recirculation cavitation is typically due to operating the pump to the left side of the pump operating curve (reduced flows) and away from the best efficiency point (BEP). The approach angle of the incoming flow does not match that of the rotating impeller inlet vane geometry. Consequently, eddy currents and turbulence are generated in between the vanes. Inside the general area of the eddy current, the velocity increases and the pressure decreases as a result. This action occurs due to the laws of conservation of energy as explained by Bernoulli’s equation and the pressure-velocity relationship. When the local pressure drops below the vapor pressure, the cavitation bubbles are formed. Recirculation cavitation is typically not caused by insufficient NPSH in the classic sense. You could have more than adequate NPSH margin and still experience recirculation cavitation because the pump is being operated away from its BEP. When this situation occurs, there is a mismatch in the flow angle as compared to the impeller inlet incidence angle. The higher the suction specific speed (NSS) of the pump impeller, the more likely recirculation cavitation is an issue. For more information on the subject of NSS, see my Pumps & Systems February 2019 article.
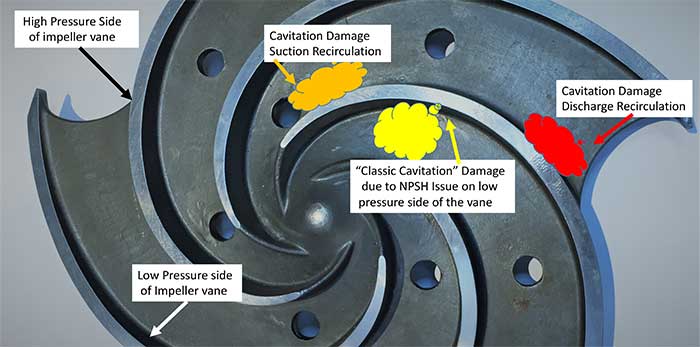
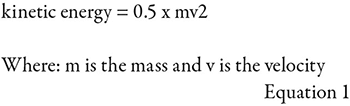