Task Force Tips is a manufacturer of firefighting equipment in the U.S. The reliability of the equipment is crucial, especially in applications in which the equipment is not frequently used. In any case, corrosion is not allowed.
The model studied in this article is the ball intake valve, commonly used on fire engines to control the flow of water into the pump. The valve’s lightweight, aluminum design has proven ideal for use on mobile equipment. The valve consists of three metals, and a patented waterway system drains off the water in the assembly after operation to minimize the corrosion risk.
In practice, however, the ball intake valve can be connected to iron or brass couplings and can remain completely filled with salt water. Put it all together, and galvanic corrosion can become a problem.
Simulation Technology
The risk of galvanic corrosion is predicted by computer simulations. A finite element analysis method is employed to solve the fundamental electrochemical equations that govern the corrosion phenomena. The simulation software consists of the following components:
- Pre-processor
- Generic and flexible CAD-import tool—Standard Tessellation Language (STL)-based
- Automatic, high-quality surface mesh
- Automated polarization curve selection
- Solver
- Robust and fast
- Supports multibody configurations and external current sources
- Post-processing
- Fully automated and configurable reporting
- Powerful visualization tool available throughout the organization
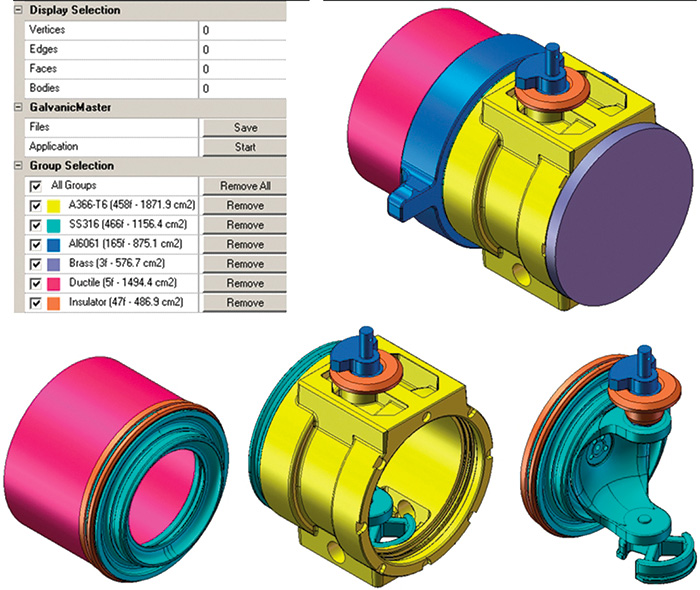
The software uses a new and unique thin film approach comprising an analysis based on a constant film thickness, a constant conductivity and a potential method approach that models the gradient of the electrolyte potential in relation to the distance to the surface. The software calculates the overlap between thin films on different bodies to ensure ion transfer between neighboring bodies with overlapping films.
The aim of this study is to predict the galvanic corrosion behavior of the ball intake valve in tap water and seawater.
Step-By-Step Modeling
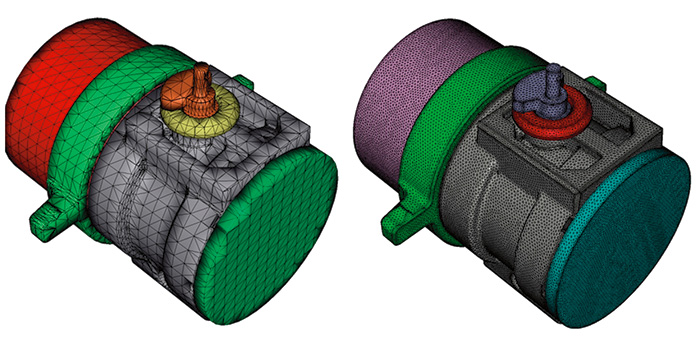
CAD-Import
In the first step, a 3-D, CAD model is loaded in the software. The user selects the different components and defines the materials. Each material group has its own color to keep a clear overview, and the total surface area for each group is listed as a reference. In this case, the assembly contains five metals (including iron and brass) and several insulating gaskets.
Defining the Boundary Conditions
In the second step, the boundary conditions need to be entered. A simple input wizard guides the user through the different steps. The environmental characteristics and electrolyte properties are defined in a first tap. For this test case, a film conductivity of 0.12 and 4.36 siemens per meter (S/m) was measured in tap water and seawater, respectively. The thin film thickness is taken to be 1 millimeter (1,000 microns) for both electrolytes.
Each metal has specific polarization behavior in the electrolyte of concern. The polarization behavior is measured by conventional electrochemical techniques, such as linear sweep voltammetry or stepwise potentiostatic method. In a second tap of the input wizard, the polarization behavior for each metal is selected from a database of polarization curves. For this study, the polarization data were measured in an electrochemical laboratory with respect to tap water and artificial seawater at 30 C.
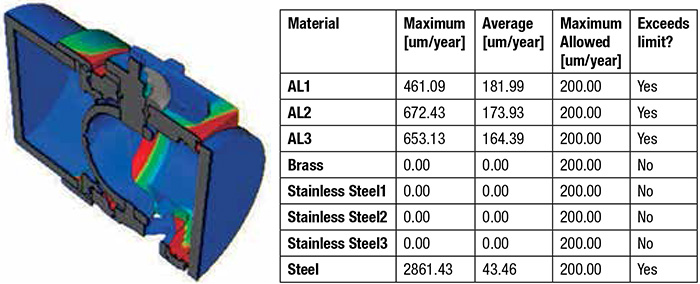
Launching the Solver
Before the calculation starts, a high-quality mesh is automatically generated based on the input STL files. This allows the accuracy of the results to increase significantly, even in confined areas.
The simulation software solves the electrochemical equations subjected to the boundary conditions established by the materials, the model and the environment. Simulation takes only a few minutes.
Analyzing the Results
The software generates a report for each simulation run. This report contains many color plots and tables that illustrate the corrosion behavior of each component. The major parameters, such as current density and corrosion rate, are given for the different components in the assembly. The galvanic corrosion behavior in tap and seawater are then easily compared, and the results can be shared within the organization.
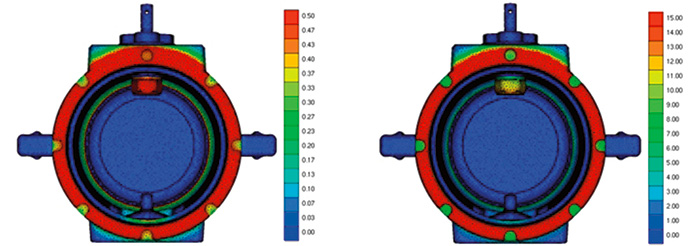
Moreover, the simulation results can be analyzed with visualization software. This tool allows users to explore the assembly in detail. In Figure 4, the brass cap was removed to look “inside” the model. The largest corrosion rates were found on the valve body, where the aluminum is in contact with the brass, and on the tip of the upper trunnion, where aluminum is close to the stainless steel of the valve ball.
The simulation results were compared with corroded equipment from the field. The aluminum trunnion and valve body’s galvanic corrosion was identified and was in line with the customer’s real-life experience.
Conclusion
The galvanic corrosion risk of a ball intake valve of fire-extinguishing equipment was investigated. The software is simple to use and allows users to determine different material combinations in a fast and effective way. In this simulation, the ball intake valve contained three metals and was capped with an iron and brass piping component. The electrochemical behavior of the metals was measured in the laboratory, and the environmental conditions were defined.
The simulation software predicted galvanic corrosion on the trunnion and valve body. The simulations are the starting point for material selection optimization. This can be accomplished by calculating the effect of finishes, coatings and alternative materials.